Passive vs Active Thermography
What is the difference between Passive vs Active Thermography?
If you ask someone what infrared is, they’d probably say “it’s the technology used in night vision goggles and gun sights”. While this might be true, there is more to it. IR produces temperature differences that are readable by cameras. In this article, we explain the basics of infrared thermography and break down the difference between passive and active thermography and its different use cases and methods.
Infrared Light, Detectors, & Cameras
Infrared light, or infrared radiation, is always around us. We just can’t see it. In fact, all objects above absolute 0 (- 273 degrees C or – 460 degrees F) emit infrared light. So not only do hot and warm objects emit infrared light, but also cold objects like a bucket of ice cubes. The amount of infrared light emitted from an object is proportional to the temperature of that object. For example, a campfire emits more IR light than a lantern and significantly more IR light than a bucket of ice.
Electromagnetic Spectrum
The electromagnetic spectrum is a term scientists use to describe the entire range of light. The following chart graphically represents the electromagnetic spectrum and shows the various forms of light, from radio waves to microwaves and x-Rays to gamma rays. What’s interesting to note is that most of the electromagnetic spectrum is invisible to us, including infrared light. From the chart, we see that only a tiny sliver of the light available in the universe is visible to us.
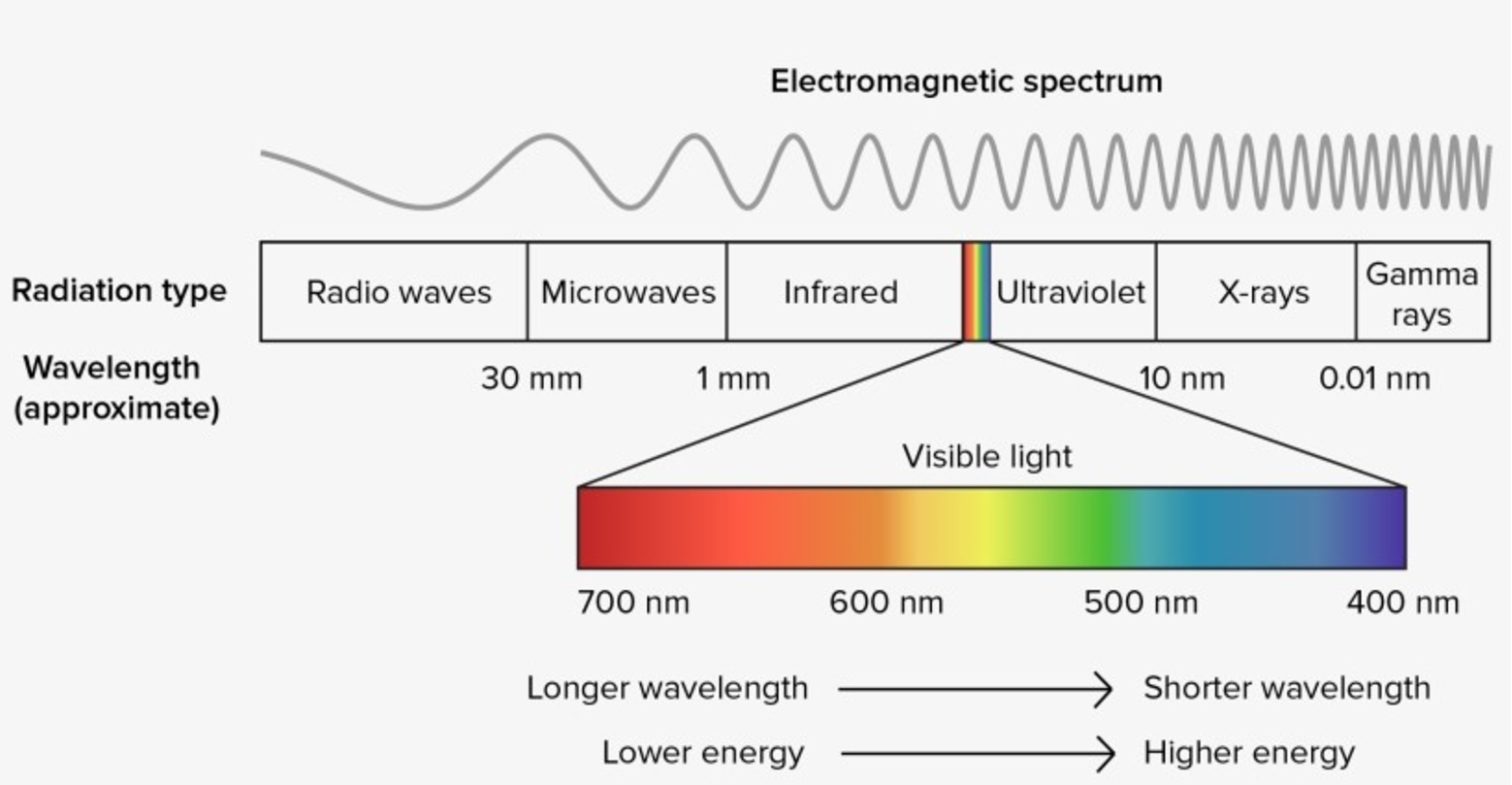
The Electromagnetic Spectrum
Also, from the electromagnetic spectrum chart, we see infrared light has a larger wavelength than visible light. Because it is only different in size, infrared light behaves similarly to visible light. For example, infrared light can be absorbed, passed through, and reflected like visible light.
While our human eye is not tuned to see infrared light, there are semiconductor materials that can sense it. By organizing these materials into a matrix of rows and columns and combining them with microelectronics, engineers have developed focal plane array (FPA) infrared detectors.
Infrared Detectors
Infrared detectors are made with materials like Amorphous Silicon, Vanadium Oxide, Indium antimonide, Indium Gallium Arsenide, and Mercury Cadmium Telluride. They come with various pixel sizes, numbers of pixels, and infrared sensing wavebands. For example, there are shortwave infrared (SWIR), midwave infrared (MWIR), and longwave infrared (LWIR) detectors that “see” infrared light in those infrared regions. Infrared FPA detectors can range in pixel resolutions from 60 x 80 (4,800 pixels) up to 1280 x 1024 (1.3 megapixels).
When integrated into a camera with an infrared lens, IR FPA detectors capture the focused infrared light that is converted into an electronic signal and presented as grayscale or colorized images. Each pixel of the infrared image represents a digitized level of infrared light. You can visually identify the image’s cold, warm, and hot areas with a corresponding grayscale or color scale palette. One can easily pick out the white-hot engine cylinders and exhaust outlets in the following infrared image of a motorcycle.
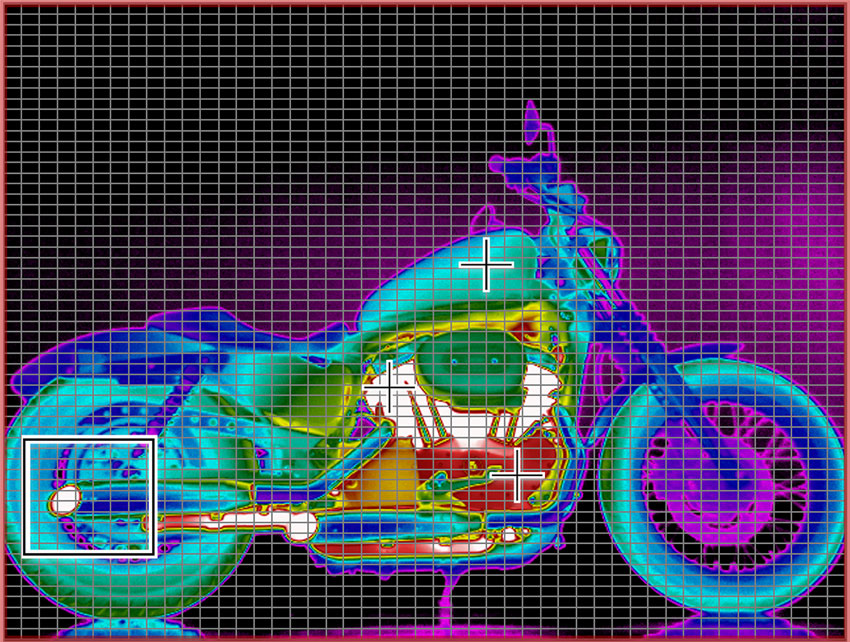
Example IR Image Motorcycle with Regions of Interest
Regions of Interest (ROI’s)
If the infrared camera is calibrated for temperature measurement, tools called regions of interest (ROI), can be applied to the live or recorded image. Images present average, minimum, and maximum temperature values. ROI’s typically include spots, lines, and areas. Because the thermal image is made up of several thousand spot measurements, temperature values can be presented from any one of the image pixels.
What is Thermography?
Thermography is the practice of using an infrared camera to see and/or measure the thermal characteristics of an object or process to assess its condition as related to temperature.
For example, during the recent Corona Virus pandemic, thermography was conducted on people to detect elevated body temperatures. Thermography was used as a pre-screening tool to identify individuals who may have a fever, a potential indicator of infection.
Another example of infrared thermography includes inspecting connections in a substation to ensure electricity flows freely. Typically, with an increase in resistance to the flow of electricity, there is a corresponding increase in heat. The increased electrical resistance could result from a loose connection, corrosion, or component failure. Regardless of the cause, increases in resistance produce rise of heat that can be seen and measured with an infrared camera.
The relationship between heat and electrical resistance can also apply to microelectronics, like looking for shorts on a printed circuit board that could shorten the life of a laptop. Other examples of thermography include:
- Looking for cold spots in buildings to identify areas of missing insulation.
- Looking for increased temperature in rotating equipment caused by the friction of a possible bearing failure.
- Observing how heat flows through the wing of an aircraft to reveal the presence of water.
What is Passive Thermography?
Any temperature distribution of the object or scene is evaluated without imposing external energy on the object. The observed temperature patterns are due solely to the inherent temperature differences.
Examples of passive thermography include the electrical substation, and microelectronics inspection discussed previously. Other examples include the inspection of a motor to be sure it is cooling properly or inspecting electrical relays to ensure they are working correctly. Each of these inspection examples relies upon the presence of a variation in temperature or variation of emitted infrared light resulting from the normal operation of the process.
What is Active Thermography?
Active thermography is applying external energy sources to an object or process to induce a variation in temperature for analysis with an infrared camera. Active thermography can be a viable nondestructive test method for objects or scenes with no naturally occurring thermal variation. In other words, no naturally occurring temperature differences exist in the object or scene.
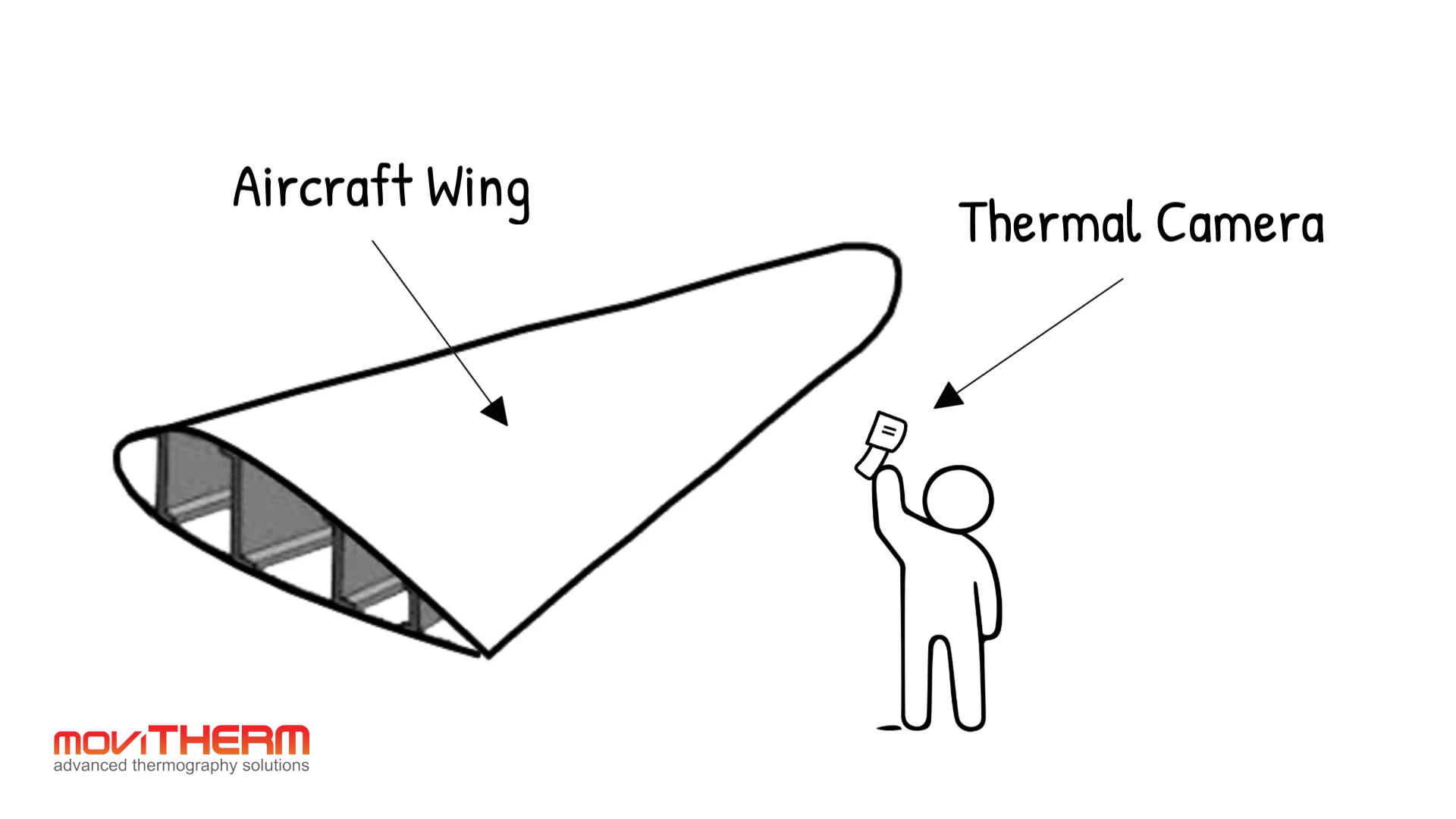
Passive Thermography: Inspecting the wing of an aircraft with a handheld thermal camera.
Suppose we want to evaluate an aircraft wing for the presence of defects. If observing the wing at a steady state with an infrared camera, we would likely see little to no thermal contrast in the resulting IR image. However, if we applied external energy to the wing, like heat from a halogen lamp, we could observe how the thermal wave from the lamp travels through the wing over time.
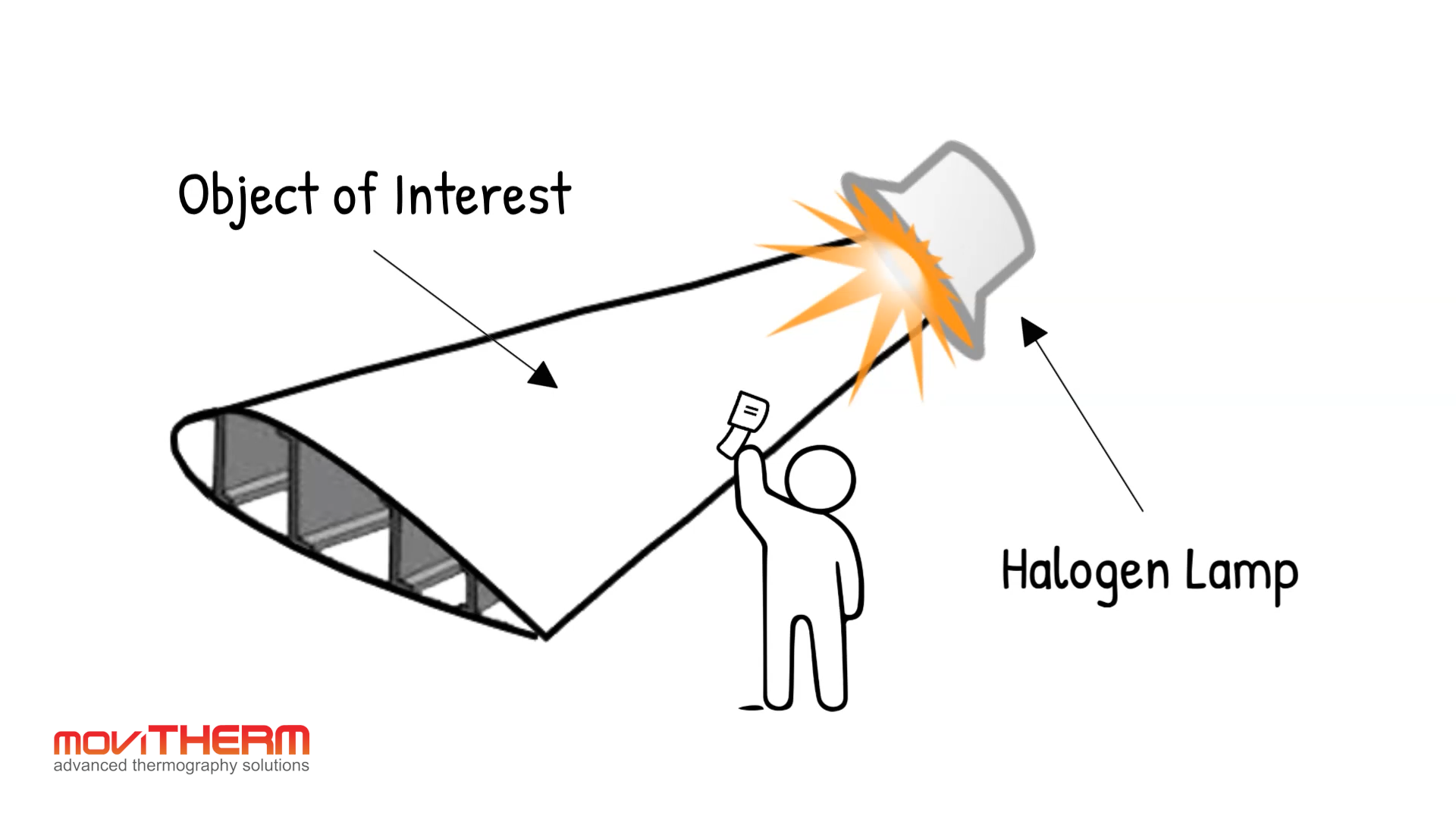
Active Thermography: Using an external excitation source to detect defects in the object of interest.
If a defect is present inside the wing, it interrupts the heat flow from the halogen lamp, causing a variation in the temperature distribution at the object’s surface. This variation is viewable with an infrared camera.
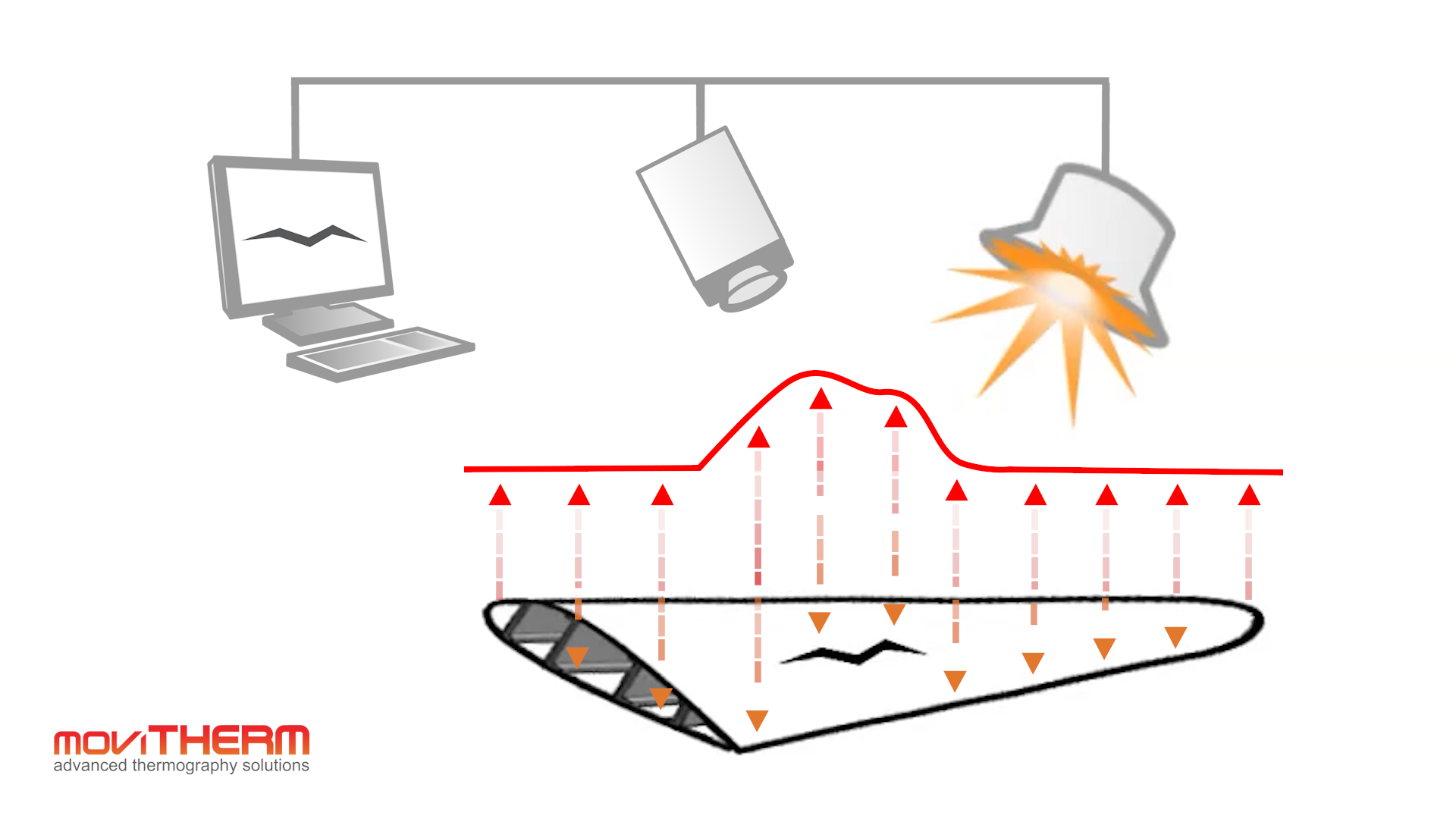
Detecting defects of an object using a thermal camera and an excitation source (halogen lamp).
One example of active thermography is using a halogen lamp to induce a thermal wave across a wing. Additional excitation sources for active thermography include:
- Xenon Flash lamps for creating short, high-intensity heat waves.
- Ultrasonic Vibration Horns induce mechanical friction at cracks and disbonds, causing heat.
- Induction coils for creating eddy currents that produce localized heat when blocked by defects.
- Programmable power supplies for introducing current into semiconductors and electronics that heat up when shorted or resisted.
- Lasers for applying pinpoint heat to small targets like microelectronics.
Inspection systems for active thermography typically use a computer to record the object’s surface temperatures as a function of time. These infrared videos are processed using software designed to “tease out” defects and anomalies that go unnoticed with passive thermography.
Various techniques for active thermography are available to conduct the most effective testing for a specific material or device. Listed below are six different types of active thermography testing techniques that are commonly used today.
Transient
Halogen light is used to create an extended heat excitation. Thermography analyzes the change in the thermal state of the target.
Flash or Pulse Thermography
Xenon light provides a short and intense excitation that is analyzed with thermography. Is well suited for materials with high thermal conductivity and shallow defects. Read our article on flash thermography to learn more.
Lock In Thermography
A periodical excitation source is synchronized with the IR camera. The software generates an amplitude and phase image to indicate the location and nature of the defect. Read our article on lock-in thermography to learn more.
Vibro Thermography
Ultrasound is used to excite the specimen. Friction between vibrating cracks creates heat signatures measured by the infrared camera. Read our article on vibro thermography to learn more.
Failure Analysis
A test specimen is excited with a periodic electrical signal and a synchronized thermal camera captures the resultant surface temperatures. Processing algorithms produce a surface map identifying localized hotspots.
Thermal Stress Analysis (TSA)
A test sample is stressed by modulating mechanical excitation with the induced infrared imagery converted into stress units.
Applications for active thermography include finding disbonds in aluminum structures like on an aircraft fuselage or seeing corrosion behind painted surfaces. Additional applications include identifying failure points in microelectronics, evaluating laser weld penetration, and visualizing wheel cracks. These are just a few of the many applications of active infrared thermography.
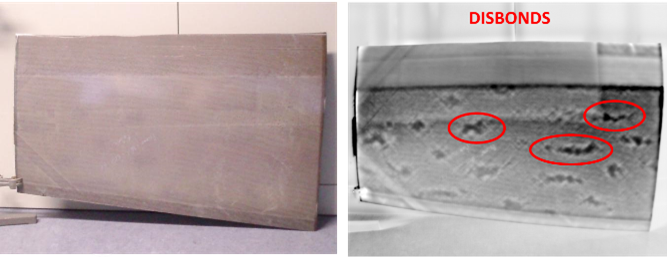
Finding Disbonds in Aluminum Structures
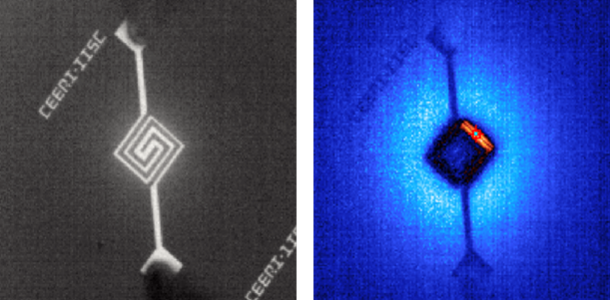
Identifying Failure Points in Microelectronics
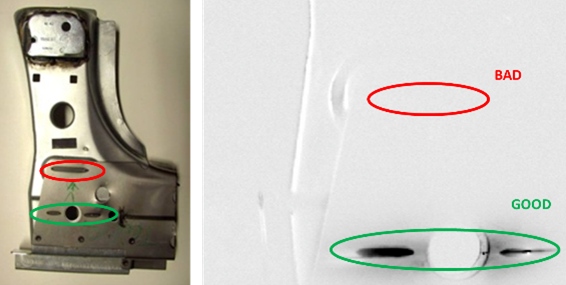
Evaluating Laser Weld Penetration
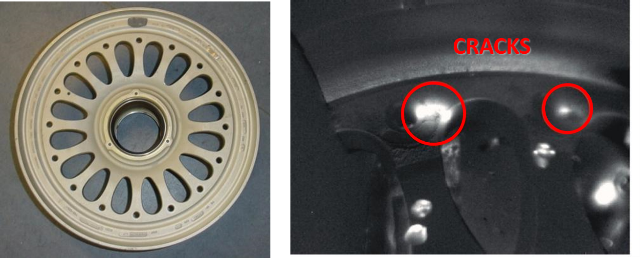
Inspecting Wheel Cracks with Infrared
Passive vs Active Thermography
Passive thermography is the use of infrared cameras or other devices for measuring the temperature of a surface. In passive thermography, the temperature of an object is measured without direct contact. The radiation emitted by an object is captured by a camera, and the temperature information is extracted from the image. Passive thermography does not require any active illumination (such as lights) or specialized equipment, making it extremely useful for inspections in dark or enclosed spaces.
Active thermography is the use of emitters to illuminate objects with infrared light and then take images using an infrared camera. The advantage of active thermography over passive thermography is that it can detect defects that might not be visible using other testing methods, including passive thermography.
Both active and passive thermographic inspections can be performed on a wide range of objects including turbines and generators, aircraft engines, electrical panels, motors, transformers and generators, HVAC systems and much more.